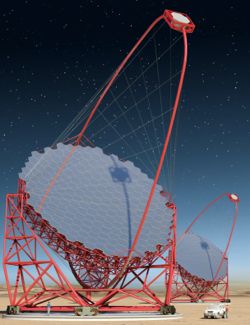
Picture yourself looking up from the base to the rooftop of a seven-story office building. The height is about the diameter of an optical mirror that CTA physicists are planning to fabricate. Another ten-story flight from the mirror’s surface takes you to a camera, attached at the apex of an elliptically-shaped structure extending perpendicularly out of the mirror, collecting rays of faint light reflecting back from the mirror beneath. The carbon-fiber structure supporting the 90-ton optical system rotates 180 degrees in mere 20 seconds. This picture roughly summarizes a CTA’s Large-Sized Telescope (LST), and there will be not just one LST, but 8, operating together with 50 Medium-Sized Telescopes (MSTs) and 72 Small-Sized Telescopes (SSTs) in two sites of the globe.
Another ten-story flight from the mirror’s surface takes you to a camera, attached at the apex of an elliptically-shaped structure extending perpendicularly out of the mirror, collecting rays of faint light reflecting back from the mirror beneath. The carbon-fiber structure supporting the 90-ton optical system rotates 180 degrees in mere 20 seconds. This picture roughly summarizes a CTA’s Large-Sized Telescope (LST), and there will be not just one LST, but 8, operating together with 50 Medium-Sized Telescopes (MSTs) and 72 Small-Sized Telescopes (SSTs) in two sites of the globe.
Cherenkov Telescope Array, or CTA, is a future ground-based gamma-ray observatory, planned to come online in 2020. The international consortium consists of over 1,000 members from 27 countries. Among the three types of telescopes, LST, targeting the lower energy range of gamma-ray spectrum, is by far the most challenging to construct, due to their sheer size.
The four-day workshop, held on January 14-17, 2014, in Kashiwa, Japan, provided a promising start toward CTA’s eventual goal. Around 80 physicists from 8 countries gathered to finalize the design of LST’s components, which include mirrors, electronics, camera, and structure, as well as to discuss physics cases and management issues.
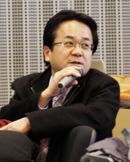
Prof. Masahiro Teshima of
ICRR, the University of Tokyo.
“We are now set to embark on the first construction phase,” says Prof. Masahiro Teshima of ICRR, the Project Integrator of CTA LST. “It is imperative that we work on the concerned parties to place our first priority on breaking ground on the foundations, as soon as our two CTA sites are settled in March.”
The crews have already completed production of photomultiplier tubes for camera, preproduction of the mirror components, and final designs of electronics and telescope interfaces. Structure is relatively more complicated, especially because it utilizes new material–carbon fiber–instead of traditional ones like steal, for its lightness and strength. The team has been working on extensive simulation studies. “Overall we are in good shape. After resolving some minor issues, we would like to order the structures in 6 months,” says Dr. Thomas Schweizer at Max-Plank Institute for Physics in Munich, the coordinator of the structure team. Production is targeted in time for the start of full scale installation in mid 2016.
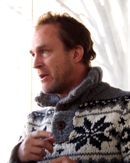
“The expectations for CTA physics from Astronomy and Cosmic Ray community are very high,” says Assoc. Prof. Hidetoshi Kubo of Kyoto University, the coordinator of electronics team. During the physics sessions on the first day, physicists explored numerous sciences that the operation of CTA will bring about, from indirect Dark Matter search, Active Galactic Nuclei survey, Gamma-ray Burst observation, evolution of the Universe, to Supernovae Remnants modeling, among others. “After KAGRA, the gravitational wave detector currently under construction, comes CTA for which we welcome interests and supports from the community.”
The CTA physics group is currently working on identifying key science projects for CTA and preparing for the analysis tools. CTA will be an open observatory, and the consortium first likes to define projects for the preparatory time during the first year of operation which will be used exclusively by the consortium itself.
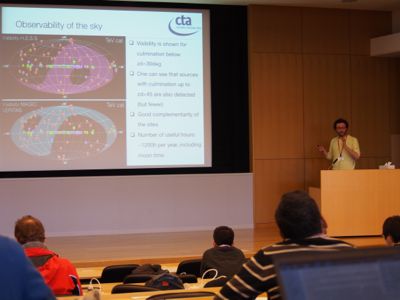
CTA sciences are very diverse, ranging from galactic and extragalactic astrophysics to fundamental physics.
“From the observation by the existing experiments we know about 50 extragalactic gamma-rays objects. With CTA sensitivity much greater, we expect to boost this number to 5 to 10 times higher,” says Junior Research Group Leader Dr. Daniel Mazin of Max Plank Institute for Physics in Munich who coordinates extragalactic physics group for CTA. Sources are mostly supermassive blackholes, that sit in the centre of galaxies, and accreting matter surrounding them. Some matter falls into blackhole, and others are ejected perpendicular to the accreting disc, forming a plasma jet where charged particles are accelerated to much higher energies. Depending on the type of particles–electrons or protons–they undergo different gamma-ray emition processes which CTA will be sensitive to detect.
“Through the very high temporal resolution and the large area of CTA we will get many photons in short time interval, and we will see the temporal evolution of the objects within seconds,” explains Dr. Mazin. “Combined with the simultaneous data from other observatories, we may be able to distinguish our models.”
Distant energetic sources of very high energy gamma-rays are also probes to the evolution of the Universe. When gamma-rays emitted from them travel through space, they interact with the background fields consisting of low energy photons from stars and dust in galaxies from all possible times in the history of the Universe. Hence, studying gamma-rays tells us what the density of background field–the amount of stars and the birthrates–has to be in terms of time evolution.
Through the imprint of the background light on the spectra of such energetic sources as active galactic nuclei (AGNs), CTA will be able to determine the Hubble constant–the measure of the scale of the Universe–independently of other methods. “If successful, CTA will be the first experiment to determine the Hubble constant by the observation of gamma-rays, which will be free from systematic errors that other methods may have,” says Dr. Mazin.
The high temporal resolution and sensitivity also allows for detection of very bright, short bursts of gamma rays, Gamma Ray Bursts (GRBs), which no ground-based Cherenkov telescopes have yet detected. “With CTA, we expect to detect 1 to 5 GRBs per year,” says Dr. Mazin. “Detecting GRBs with CTA will largely improve our understanding of their nature. Moreover, together with distant AGNs, GRBs can greatly help us understand the early universe especially thanks to low energy threshold of CTA, allowing detection of GRBs from distant universe up to a high redshift of z=6 or even beyond.”
CTA will also shed light on the century-old problem of the origin of cosmic rays–where and how high energy particles are produced, and how they are accelerated. “With full array CTA observations, we will be able to solve the problem of production and acceleration of high energy galactic cosmic rays, and also determine their maximum energy,” says Dr. Pratik Majumdar of Saha Institute of Nuclear Physics, India.
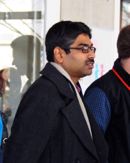
Physicists will be looking at galactic supernova remnants (SNRs), an end product of a violent explosion when a star dies, as a promising source of high energy cosmic rays. When stars explode, they throw out a large amount of matter, colliding into the surrounding interstellar medium at very high speeds. This process creates “shock” waves, in which particles get accelerated to very high energies.
“The easiest way to study these high energy particles is through the radiation the particles produce when they are accelerated. The produced photons are still up scattered to higher energies through interactions with the electrons which produce the photons in the first place. So from the study of very high energy gamma rays, you can study acceleration of charged particles from SNRs,” explains Dr. Majumdar.
There are also large hopes for unexpected. CTA will be at the forefront of elusive dark matter search, with unprecedented sensitivity to gamma-rays which candidate astrophysical dark matter particles may produce.
Around 150 LST collaborators are working close together across the globe. Components will be assembled and tested, and the collaborators will meet again in 6 months to report their progress. Holding the conference in Japan “was a good opportunity for the collaboration to meet students here who do the actual work for the team and to have them present their work,” adds Prof. Kubo. For oversea collaborators, it was also an opportunity to experience a taste of oriental culture, with an excursion to observe Sumo wrestling match on the last day of the workshop.
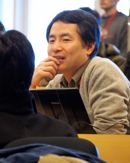
